Surprising Twist To Photosynthesis: Scientists Swap Key Metal Necessary For Turning Sunlight Into Chemical Energy
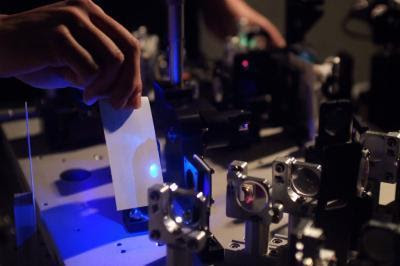
Photosynthesis is a remarkable biological process that supports life on earth। Plants and photosynthetic microbes do so by harvesting light to produce their food, and in the process, also provide vital oxygen for animals and people।
Now, a large, international collaboration between Arizona State University, the University of California San Diego and the University of British Columbia, has come up with a surprising twist to photosynthesis by swapping a key metal necessary for turning sunlight into chemical energy.
The team, which includes: ASU scientists Su Lin, Neal Woodbury, Aaron Tufts and James P. Allen; UBC colleagues J. Thomas Beatty, Paul R. Jaschke, Federico I. Rosell and A. Grant Mauk; Mark Paddock, UCSD; Haiyu Wang, Jilin University, China, described their findings in the May 11 early online edition of the Proceedings of the National Academy of Sciences.
In the heart of every green leaf are pigments called chlorophyll, which not only give most plants their color, but also along with the yellow and orange carotenoid pigments, are key molecules that harvest light across the spectrum.
In all plant chlorophylls, only one particular metal, magnesium, is held tightly within the molecule's center.
During photosynthesis, plants have two photosystems that work in tandem: photosystem I and photosystem II. To peer at the inner workings of photosynthesis, the team used a hardy, well-studied, photosynthetic bacterium called Rhodobacter sphaeroides. An organism similar to this purple bacterium was likely one of the earliest photosynthetic bacteria to evolve. The purple bacteria possess a simplified system similar to photosystem II.
The center stage of photosynthesis is the reaction center, where light energy is funneled into specialized chlorophyll binding proteins. The research team had previously demonstrated that the movement of the reaction center proteins during photosynthesis facilitates the light-driven movement of electrons between molecules in the reaction center, helping the plant or bacteria to harness light energy efficiently even if conditions aren't optimal. Every time the team introduced disruptions into this electron pathway, the proteins were able to compensate by moving and energetically guiding the electrons through their biological circuit.
"One of our research strategies is to introduce mutations into the bacteria and study how these affect the energy conversion efficiency of the reaction center," said Su Lin, PhD, senior researcher at ASU's Department of Chemistry & Biochemistry and Biodesign Institute, and lead author of the study. "Carefully-designed aberrations provide extensive information about the normal mechanism of energy conversion in reaction centers, just like studying a disease clarifies the parameters of health for the involved biochemical pathways and tissues. From this, we can learn a lot about the most basic mechanisms of photosynthesis."
The reactions that convert light to chemical energy happen in a millionth of a millionth of a second, which makes experimental observation extremely challenging. A premier ultrafast laser spectroscopic detection system that Lin has built, with the sponsorship of the National Science Foundation, acts like a high-speed motion picture camera. It splits the light spectrum into infinitesimally discrete slivers, allowing the group to capture vast numbers of ultrafast frames from the components of these exceedingly rapid reactions. These frames are then mathematically assembled, allowing the group to make a figurative 'movie' of the energy transfer events of photosynthesis.
The current research study began when Paul R. Jaschke, a graduate student with professor J. Thomas Beatty in the Department of Microbiology and Immunology at the University of British Columbia, discovered a mutant that replaced the magnesium metal found in the reaction center with zinc.
"We initially thought this reaction center was non-functional," said Beatty. "We were forced to think in new ways to explain the surprising results, which led to some nice insight."
Lin carefully measured the light absorption spectra for the naturally occurring magnesium reaction center and compared it to the mutant reaction center that was replaced with zinc bacteriochlorophylls. She found that, though the zinc-coordinated reaction center is comprised of six bacteriochlorophylls, changing their structure to a configuration similar to that used in plant photosystem I reaction centers, surprisingly, the data from the reaction kinetics and the energy conversion efficiency were almost identical to the magnesium containing reaction center.
"Amazingly, the reaction center still works with essentially the same physical chemical properties as the normal system," said Neal Woodbury, deputy director of the Biodesign Institute. "This was a real puzzle when Su first did these measurements, but she was able to figure out why."
"The electron transfer driving force can be determined by either the properties of the metal cofactors themselves or through their interaction with the protein," said Lin. "In the case of the zinc reaction center, the driving force is regulated through the coordination of the metal."
"Once again, biology shows its resilience so that changes in one area are compensated by changes in others and the protein's ability to dynamically adjust," said Woodbury.
The results may enable researchers to explore a deeper understanding of the structure, function, and evolution of photosynthesis reaction centers in photosystems I and II. Of particular interest, are studies that focus on the interaction between chlorophylls and protein, which differs in naturally occurring reaction center variants. The team may also conduct future experiments to understand the metal substitution limitations of the reaction center and track the protein movements that may be occurring in the reaction center that helps to optimize photosynthesis.
Their results may have long-term practical applications for the development of next-generation solar cells, which could, through biomimicry of photosynthesis, greatly boost the energy efficiency compared with current technology. The robustness of the natural system may offer some useful lessons for engineers trying to improve on current technologies, and bring the costs of solar panels down to the average consumer.
Woodbury has proposed that there might be a way to increase the flexibility of the system used in organic solar cells by incorporating solvents that move on a variety of time scales that could "tune" the molecules to work in a wider variety of conditions.
Journal reference:
Su Lin, Neal Woodbury, Aaron Tufts and James P. Allen, J. Thomas Beatty, Paul R. Jaschke, Federico I. Rosell and A. Grant Mauk; Mark Paddock, Haiyu Wang. Electron transfer in the Rhodobacter sphaeroides reaction center assembled with zinc bacteriochlorophyll. Proceedings of the National Academy of Sciences, 2009; DOI: 10.1073/pnas.0812719106
The team, which includes: ASU scientists Su Lin, Neal Woodbury, Aaron Tufts and James P. Allen; UBC colleagues J. Thomas Beatty, Paul R. Jaschke, Federico I. Rosell and A. Grant Mauk; Mark Paddock, UCSD; Haiyu Wang, Jilin University, China, described their findings in the May 11 early online edition of the Proceedings of the National Academy of Sciences.
In the heart of every green leaf are pigments called chlorophyll, which not only give most plants their color, but also along with the yellow and orange carotenoid pigments, are key molecules that harvest light across the spectrum.
In all plant chlorophylls, only one particular metal, magnesium, is held tightly within the molecule's center.
During photosynthesis, plants have two photosystems that work in tandem: photosystem I and photosystem II. To peer at the inner workings of photosynthesis, the team used a hardy, well-studied, photosynthetic bacterium called Rhodobacter sphaeroides. An organism similar to this purple bacterium was likely one of the earliest photosynthetic bacteria to evolve. The purple bacteria possess a simplified system similar to photosystem II.
The center stage of photosynthesis is the reaction center, where light energy is funneled into specialized chlorophyll binding proteins. The research team had previously demonstrated that the movement of the reaction center proteins during photosynthesis facilitates the light-driven movement of electrons between molecules in the reaction center, helping the plant or bacteria to harness light energy efficiently even if conditions aren't optimal. Every time the team introduced disruptions into this electron pathway, the proteins were able to compensate by moving and energetically guiding the electrons through their biological circuit.
"One of our research strategies is to introduce mutations into the bacteria and study how these affect the energy conversion efficiency of the reaction center," said Su Lin, PhD, senior researcher at ASU's Department of Chemistry & Biochemistry and Biodesign Institute, and lead author of the study. "Carefully-designed aberrations provide extensive information about the normal mechanism of energy conversion in reaction centers, just like studying a disease clarifies the parameters of health for the involved biochemical pathways and tissues. From this, we can learn a lot about the most basic mechanisms of photosynthesis."
The reactions that convert light to chemical energy happen in a millionth of a millionth of a second, which makes experimental observation extremely challenging. A premier ultrafast laser spectroscopic detection system that Lin has built, with the sponsorship of the National Science Foundation, acts like a high-speed motion picture camera. It splits the light spectrum into infinitesimally discrete slivers, allowing the group to capture vast numbers of ultrafast frames from the components of these exceedingly rapid reactions. These frames are then mathematically assembled, allowing the group to make a figurative 'movie' of the energy transfer events of photosynthesis.
The current research study began when Paul R. Jaschke, a graduate student with professor J. Thomas Beatty in the Department of Microbiology and Immunology at the University of British Columbia, discovered a mutant that replaced the magnesium metal found in the reaction center with zinc.
"We initially thought this reaction center was non-functional," said Beatty. "We were forced to think in new ways to explain the surprising results, which led to some nice insight."
Lin carefully measured the light absorption spectra for the naturally occurring magnesium reaction center and compared it to the mutant reaction center that was replaced with zinc bacteriochlorophylls. She found that, though the zinc-coordinated reaction center is comprised of six bacteriochlorophylls, changing their structure to a configuration similar to that used in plant photosystem I reaction centers, surprisingly, the data from the reaction kinetics and the energy conversion efficiency were almost identical to the magnesium containing reaction center.
"Amazingly, the reaction center still works with essentially the same physical chemical properties as the normal system," said Neal Woodbury, deputy director of the Biodesign Institute. "This was a real puzzle when Su first did these measurements, but she was able to figure out why."
"The electron transfer driving force can be determined by either the properties of the metal cofactors themselves or through their interaction with the protein," said Lin. "In the case of the zinc reaction center, the driving force is regulated through the coordination of the metal."
"Once again, biology shows its resilience so that changes in one area are compensated by changes in others and the protein's ability to dynamically adjust," said Woodbury.
The results may enable researchers to explore a deeper understanding of the structure, function, and evolution of photosynthesis reaction centers in photosystems I and II. Of particular interest, are studies that focus on the interaction between chlorophylls and protein, which differs in naturally occurring reaction center variants. The team may also conduct future experiments to understand the metal substitution limitations of the reaction center and track the protein movements that may be occurring in the reaction center that helps to optimize photosynthesis.
Their results may have long-term practical applications for the development of next-generation solar cells, which could, through biomimicry of photosynthesis, greatly boost the energy efficiency compared with current technology. The robustness of the natural system may offer some useful lessons for engineers trying to improve on current technologies, and bring the costs of solar panels down to the average consumer.
Woodbury has proposed that there might be a way to increase the flexibility of the system used in organic solar cells by incorporating solvents that move on a variety of time scales that could "tune" the molecules to work in a wider variety of conditions.
Journal reference:
Su Lin, Neal Woodbury, Aaron Tufts and James P. Allen, J. Thomas Beatty, Paul R. Jaschke, Federico I. Rosell and A. Grant Mauk; Mark Paddock, Haiyu Wang. Electron transfer in the Rhodobacter sphaeroides reaction center assembled with zinc bacteriochlorophyll. Proceedings of the National Academy of Sciences, 2009; DOI: 10.1073/pnas.0812719106
Comments